Could the seemingly simple act of a "laser bounce" hold the key to groundbreaking advancements across a multitude of scientific and technological fields? The reflective interaction of light, when meticulously controlled and analyzed, presents a powerful tool for measurement, detection, and manipulation, offering solutions that were once confined to the realm of science fiction.
From the subtle shifts in a beam's trajectory to the intricate patterns it forms upon interaction with matter, the phenomenon of a "laser bounce" provides invaluable insights. It transcends the limitations of traditional methods, allowing for non-destructive testing, incredibly precise distance measurements, and the ability to probe the very fabric of materials. It is the embodiment of elegant simplicity a light beam meeting its match and relaying its secrets back to the observer. This is the core concept we explore here.
The potential applications span an astonishing range. Medical diagnostics can be revolutionized through advanced imaging techniques. Industrial processes can benefit from enhanced quality control and automated inspection. And, in the realm of fundamental research, the study of "laser bounce" provides access to new understanding of material properties, even enabling the exploration of extreme conditions. The essence of this fascinating topic is the interaction of light with various substances, and its unique application in diverse fields.
Aspect | Details |
---|---|
Fundamental Principle | The reflection, refraction, and scattering of a laser beam when it interacts with a surface or medium. This interaction provides information about the characteristics of that surface or medium. |
Key Properties Exploited |
|
Measurement Techniques |
|
Applications |
|
Advantages |
|
Challenges |
|
Future Trends |
|
Related Technologies |
|
The initial exploration of "laser bounce" techniques was driven by the need for precise measurements. Early applications centered on distance determination, using the time it takes for a laser pulse to reflect back from a target. This Time-of-Flight (ToF) method, pioneered in the early decades of laser development, provided a revolutionary advance over traditional surveying methods. These early forays into the science established fundamental principles. By analyzing the subtle shifts in the reflected light, scientists could calculate the distance with unprecedented accuracy, leading to applications in surveying, mapping, and even radar systems. The very essence of "laser bounce" reveals the core principle of light's interaction, and from this, various applications have been created.
One of the most significant contributions in the field of "laser bounce" lies in the development of Light Detection and Ranging (LiDAR) technology. LiDAR utilizes a laser to scan a surface, creating a detailed 3D map. The precise measurement of the time it takes for the laser light to return to a sensor allows for highly accurate distance measurements, even over vast areas. This technology has become indispensable in applications such as autonomous vehicles, environmental monitoring, and archaeological surveys. Imagine the precision of autonomous driving, which would not have been possible without the "laser bounce" technology.
Interferometry, a related technique, further enhances the power of "laser bounce." This method uses the interference patterns created when two or more laser beams interact. By carefully analyzing these patterns, incredibly minute changes in distance, displacement, or refractive index can be detected. Interferometry finds use in a broad spectrum of fields, from precision manufacturing of microchips to the study of gravitational waves. The exquisite sensitivity of interferometry allows for the detection of changes that are far beyond the capacity of human perception. It represents a very sensitive application of the principle of the "laser bounce."
The application of "laser bounce" principles extends to advanced medical imaging. Optical Coherence Tomography (OCT) is a non-invasive imaging technique that uses "laser bounce" to create high-resolution cross-sectional images of biological tissues. OCT allows physicians to visualize structures beneath the surface, which makes it invaluable for diagnosing a wide range of conditions, particularly in ophthalmology, cardiology, and dermatology. Through the principle of light scattering and reflection, the technique provides information on internal structures. This application underscores the impact of "laser bounce" technologies on healthcare and medical science.
The industrial sector has also benefited significantly from advancements in "laser bounce" technology. Laser scanners are now used for quality control in manufacturing. The ability to rapidly and accurately scan objects enables automated inspection of parts, detecting defects and ensuring that products meet required specifications. Laser-based measurement systems also facilitate precise alignment and positioning of machinery. These techniques improve efficiency, reduce waste, and ultimately lead to higher-quality products. The advantages of the "laser bounce" in the industrial sector are clear: more precision, better control, and greater accuracy.
Spectroscopy represents another critical aspect of "laser bounce" applications. This technique uses the interaction of light with matter to identify the composition and characteristics of a substance. When a laser beam interacts with a material, the light may be absorbed, transmitted, or scattered. By analyzing the spectrum of light reflected or transmitted, scientists can identify the material's chemical and physical properties. The result of this "laser bounce" gives unique insights into the materials. This technique finds uses in various areas, from environmental monitoring to security applications.
Environmental monitoring is another area where "laser bounce" technology plays a crucial role. LiDAR systems are used to measure air pollution levels by detecting the presence of specific gases in the atmosphere. The technology is also used in the study of climate change by measuring the height of clouds and ice sheets. Other techniques, such as Raman spectroscopy, can analyze the chemical composition of pollutants in the air or water. By employing "laser bounce" in this manner, we gain a deeper understanding of our environment.
The field of art and archaeology has also embraced "laser bounce" technologies. Laser scanners are used to create highly detailed 3D models of artifacts and historical sites. This allows for preservation and study of the past with minimal impact. Furthermore, the non-destructive nature of these techniques is invaluable when working with fragile and valuable objects. Techniques derived from the principle of "laser bounce" allow us to study things without even touching them.
Looking ahead, the future of "laser bounce" seems exceptionally bright. There's continuous development in laser sources, sensors, and data processing techniques. The integration of artificial intelligence and machine learning is expected to play an increasingly significant role in the analysis of data. As a result, we can anticipate more refined and sophisticated applications in fields such as medicine, manufacturing, and environmental science. This growth is supported by ongoing developments in the science of the "laser bounce."
Research and development continue, constantly pushing the boundaries of whats possible. From the development of new materials with tailored reflective properties to advanced algorithms for interpreting complex data, innovation is driving the evolution of laser bounce technology. These advances will lead to new discoveries and applications in the years to come. The future looks bright, and it rests on the principle of the "laser bounce."
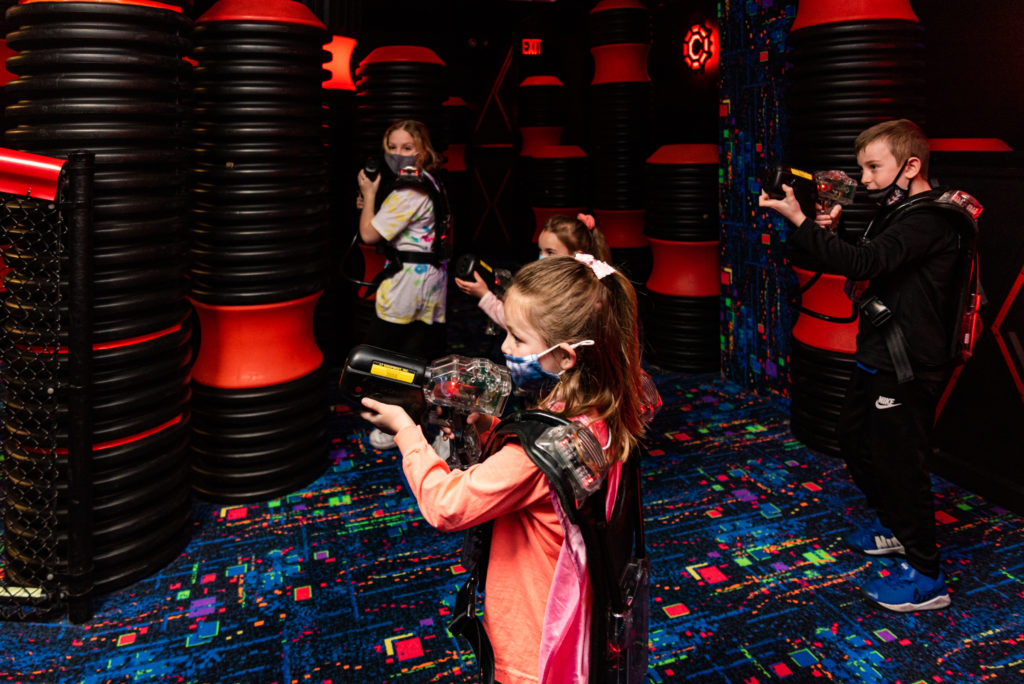
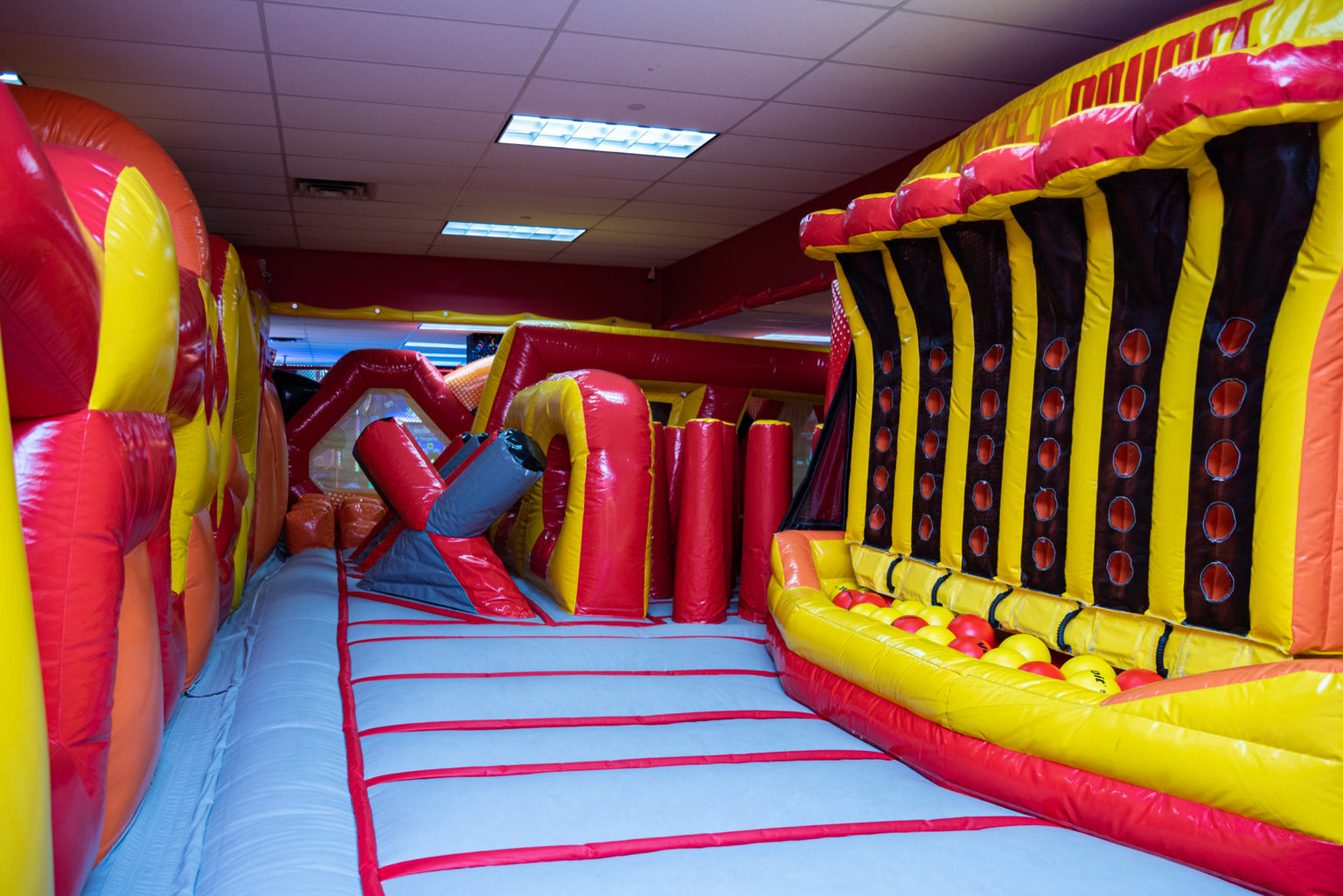
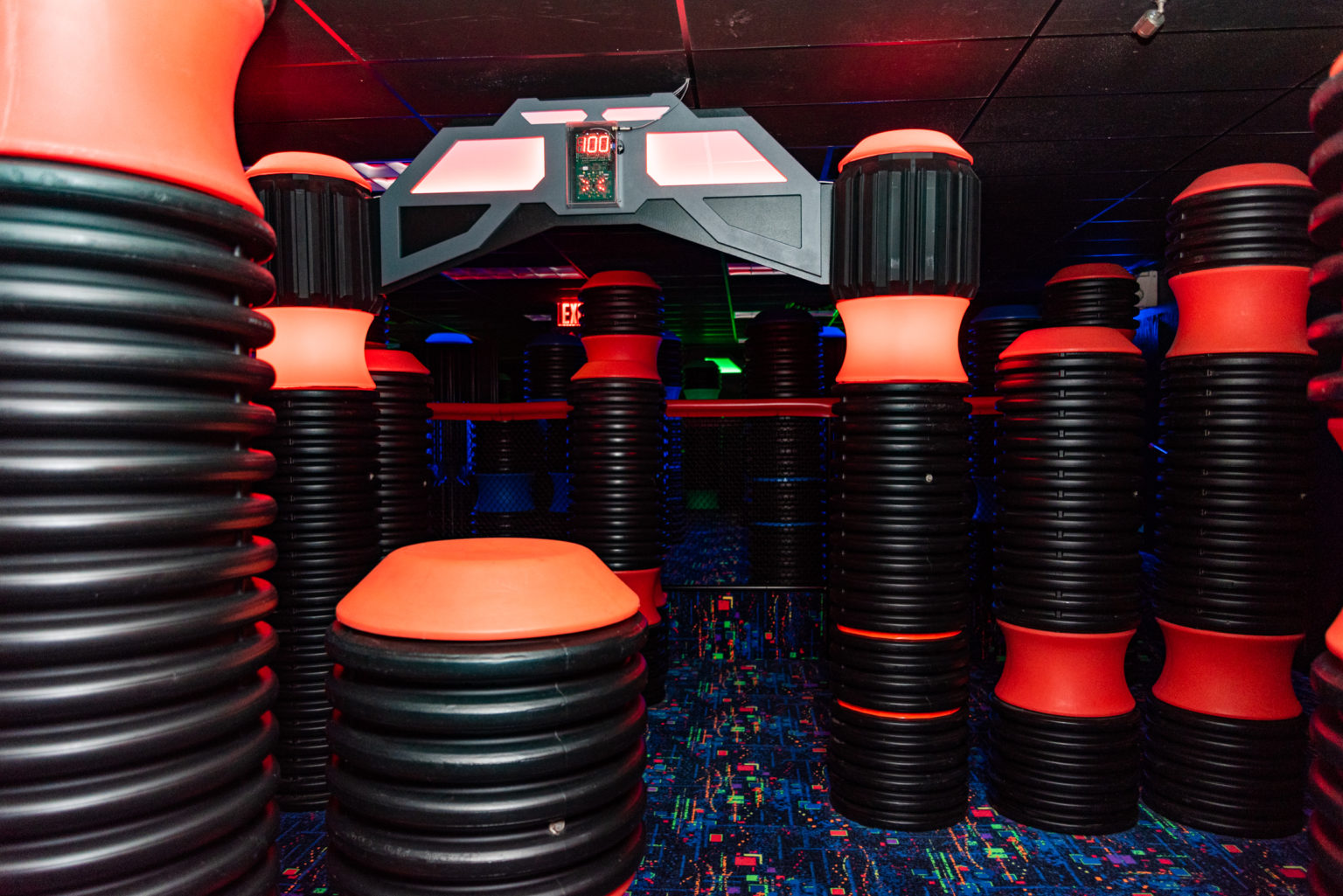